Magnets are ubiquitous in modern life, used in motors, generators, wind turbines, steel foundries, toys, and all sorts of technological equipment. They are also essential for several distinct classes of devices, including Magnetic Resonance Imaging scanners.
Different types of coils used in Magnetic Resonance Imaging and their simulation with SIMULIA Opera® are introduced in this blog post. Aspects of superconducting coil simulation and the shielding of the strong magnetic fields to minimize human exposure will also be discussed.
Coils used in Magnetic Resonance Imaging (MRI)
Magnetic Resonance Imaging, or MRI, was first used to produce images in 1980. It uses a variety of magnetic coils and fields to produce detailed internal images of the body, and it is a vital component of modern medical care.
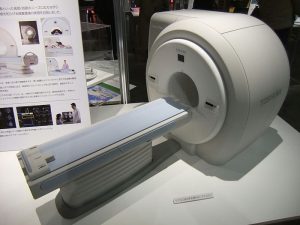
Different coils and fields are required for the operation of an MRI scanner:
- A strong, highly homogeneous, axial (z-directed) DC magnetic field is produced over the image volume causing protons in the body to align either transverse or parallel to the field.
- The required position of the image slice is selected using the z-gradient coils, which give a small linear variation to the main DC field, causing the resonant frequency of the protons to vary as a function of position in z.
- A high-frequency coil-set generates an RF pulse sequence, which excites those protons that are resonant at that frequency, causing them to spin out of equilibrium. These protons will be in a slice that depends on the frequency and total field.
- Following the RF pulse, z-directed gradient fields are applied along the x and y directions, with defined frequency and phase distributions. As the protons realign to the magnetic field, the x- and y-gradient fields modify the RF that they radiate in both, frequency and phase, allowing localization in x and y within the slice.
- Image contrast between tissues is provided by the density and environment of the protons, and by their relaxation time from the excited state.
MRI machines have seen a continuous development of requirements:
- Higher DC fields increase the proton resonant frequency, which enables the choice of higher resolutions and/or shorter scan times, leading to better diagnostics and patient experience.
- Open-bore, dipolar or Halbach magnet technology can improve patient experience, broaden the range of patients able to undergo diagnostic procedures, and enable device portability.
- Cost and manufacturing improvements to enable greater access to healthcare.
Each of these requirements presents its own design challenges, which SIMULIA can help engineers to understand and overcome. SIMULIA Opera can be used to simulate the main, shield and gradient coils in an MRI system, as well as help design passive shielding to keep the fields in public spaces at no more than about 10 times Earth’s field (25 – 65 microTesla). Typically, the field at the center of a modern MRI is about 60,000 times stronger than Earth’s field! The Opera formulation of the electromagnetic fields achieves accuracy in the imaging volume that exceeds parts per million – necessary for successful MRI design and correction.
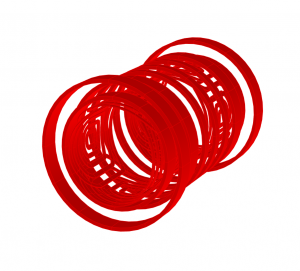
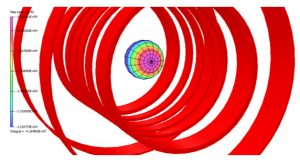
Superconducting Coils and Quench Simulation
While the gradient coils are usually normally conducting wires or sheets, the main and shield coils are most often superconducting. Low Temperature Superconducting (LTS) coils usually operate at around 4 K, carrying large currents without resistance to produce the intense magnetic fields required for high MRI resolution. If part of the superconductor becomes normal conducting, the coil will ‘quench’ – the normal conducting region will become resistive and generate heat, spreading the quench through the coil. In fact, part of the MRI magnet being prepared for full operation is the purposeful quenching or “training” of the magnet several times, which allows it to operate routinely with design parameters.
SIMULIA Opera can simulate a superconducting quench event, as well as the appropriate mitigation measures. Since a quench event can be triggered by a relatively small amount of applied heat, it is also important to design a thermal shielding system for the gradient coils, which are operating with periodic waveforms and hence induce eddy currents. This can be simulated using the Opera multiphysics options, enabling designers to conduct studies virtually, avoiding the tens of thousands of Euros of costs associated with an unplanned quench event.
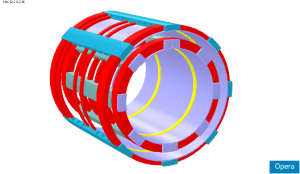
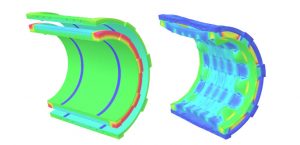
Control circuits, that can respond when a quench is detected, are an important part of the quench protection system. The idea is to protect the magnet by safely dissipating the stored energy, for instance into shunt resistors. This helps to limit the localized temperature rise. Intentionally quenching the other coils on the circuit to dissipate energy through their own shunt resistors and prevent localized damage is another sensible precaution. Finally, the cryogenic helium may be vented as a safety measure.
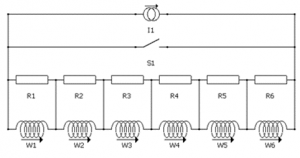
Simulation of MRI Shielding
Mandatory limits exist for magnetic fields in uncontrolled areas around MRI machines, to prevent exposure to high fields that may present a hazard – for instance, interfering with the operation of pacemakers. Stray fields from MRI machines are not only shielded with the MRI magnet’s own active shielding coils but also by ferrous materials around the chamber walls, which can ensure the containment of potentially hazardous fields within controlled areas.
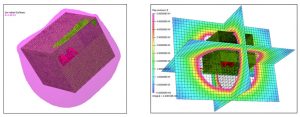
The shield, as well as other magnetic material nearby, can influence the MRI main field homogeneity – essential for machine operation. This must be corrected and maintained at the MRI location.
In addition, MRI machines are often upgraded with a higher field magnet, or re-sited and reused, meaning that shielding must be redesigned. Magnetic shields are both expensive and heavy, simulation using SIMULIA Opera allows the optimal amount of material to be used to ensure safe operation.
Opera uses a proprietary representation of the shield (Opera Thin Plate boundary condition) to avoid challenges associated with modelling and solving high aspect ratio structures. This model incorporates parameters defining material properties, shield thickness and optionally laminations in the shield. It is a relatively simple task to access the parameters using the Opera Optimizer to assist designers in automatically specifying a shield to meet the environmental considerations.
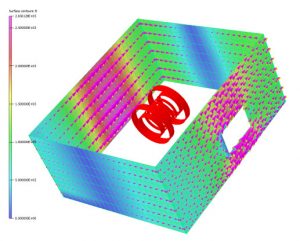
Summary
As mentioned at the beginning of this article, the requirements for MRI scanners have evolved greatly over the last 50 years, and we don’t expect that rate of development to change any time soon. We’ll be helping designers, engineers and installers to look at higher fields, new materials (such as High Temperature Superconductors) and MRI-guided therapy devices, whilst simultaneously considering the concerns of today, such as sustainability and affordable access to healthcare.
SIMULIA offers an advanced simulation product portfolio, including Abaqus, Isight, fe-safe, Tosca, Simpoe-Mold, SIMPACK, CST Studio Suite, XFlow, PowerFLOW, and more. The SIMULIA Community is the place to find the latest resources for SIMULIA software and to collaborate with other users. The key that unlocks the door of innovative thinking and knowledge building, the SIMULIA Community provides you with the tools you need to expand your knowledge, whenever and wherever.