A common chemistry class experiment is to connect each end of a battery to a pencil and stick the pencils in salty water. Tiny bubbles will appear at the pencil ends: oxygen gas on one side, and hydrogen on the other — a process known as electrolysis.
The idea behind a fuel cell is to reverse this process: to generate electricity from oxygen and hydrogen gas. This electricity can be used to drive cars, power mobile phones and laptops, provide electricity in off-grid locations, and even explore space with just one by-product: pure water. This provides an eco-friendly way of energy consumption that can run non-stop simply by adding more fuel, unlike batteries which often take hours or days to recharge fully.
Figure 1 Schematic of a fuel cell. Hydrogen (H2) dissociates at the Anode (left). Resulting protons (H+) diffuse through the Proton-Exchange Membrane (PEM) to the Cathode (right), where they react with oxygen (O2) to form water (H2O).
BIOVIA apprentices Sunil Kumar and Anshul Singhal have taken an atomistic look at the workings of fuel cells, in particular at what goes on inside the polymer membrane component. This membrane serves as a channel for proton transport and acts as a barrier for gas crossover. They presented their results at a recent CECAM conference MD@60 in Bangalore, India, and received an award from the American Institute of Physics
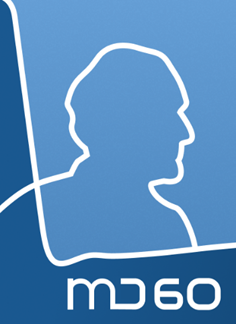
The conference marked the 60th anniversary of the seminal paper by Aneesur Rahman “Correlations in the Motions of Atoms in Liquid Argon” (Phys. Rev. 136, A405, 1964) that laid the foundations for what is now known as the Molecular Dynamics (MD) method. Thanks to the ever-growing power of computational resources, and the development of faster algorithms and more sophisticated models, today’s MD simulations can be applied to increasingly complex systems, such as proton-exchange membranes (PEMs).
An essential consideration for fuel cell applications is their lifetime. Typical PEM fuel cells have a lifespan of 40,000 to 80,000 hours of operation. Engineers and material scientists are continually making advancements to extend their durability. Gas crossover forms one of the challenges; this is not only wasteful fuel loss, but can also cause unwanted reactions to occur, which in turn can degrade the polymer and impact the proton conductivity, and ultimately the operation of the fuel cell.
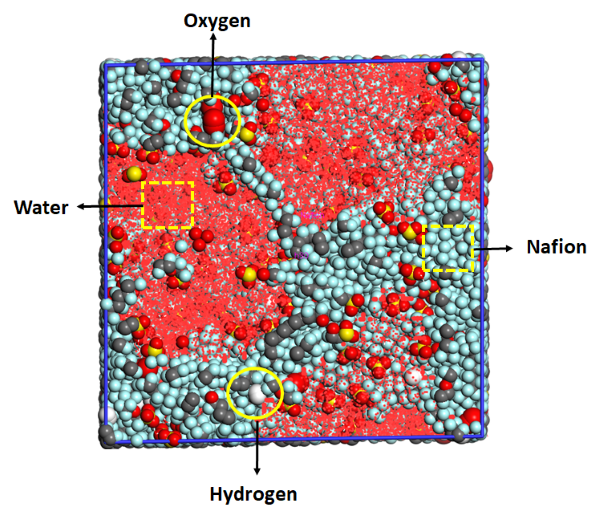
Figure 2 Molecular model of a hydrated Nafion membrane loaded with a gas impurity.
Anshul has studied the gas crossover through Nafion PEMs as a function of the hydration level, by calculating the diffusivity and solubility from MD simulations using BIOVIA Materials Studio. The hydration level increases the diffusion rates of gases like oxygen and hydrogen. Simulations with the COMPASSIII forcefield confirmed this trend quantitatively for both compounds, as well as for water itself. Solubility, on the other hand, is expected to decrease as a function of water concentration, and again the simulations predicted this trend correctly. Together, they determine the permeability, an important design parameter for membranes.
When oxygen reaches the anode side, it can react with hydrogen radicals to form hydrogen peroxide. This reactive compound can attack the side chains of the Nafion polymer, which are crucial in proton transport. Sunil has investigated this degradation mechanism by combining quantum mechanics, MD and Monte Carlo methods in Materials Studio. Firstly, reaction energies and activation barriers were calculated using the reaction pathway method FlexTS. Then MD simulations were executed on a Nafion-peroxide system, where reactions can occur periodically. Finally, the event sequence was projected on a physical timeline using kinetic Monte Carlo, to allow estimation of reaction rate coefficients.
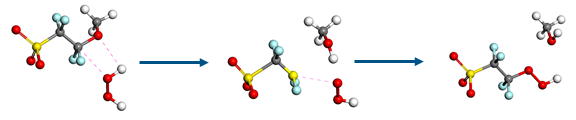
Figure 3 Cleavage of a Nafion side chain by hydrogen peroxide.
Together the projects provide us with a better understanding of membrane materials for fuel cells. The results can be used to drive mesoscale simulations of proton conductivity in Nafion membranes, and study the effect of polymer degradation on transport properties. In turn these results feed into more macroscopic models developed at other 3DS brands.
If you are interested in joining the Dassault Systèmes internship program, click here to see available positions.